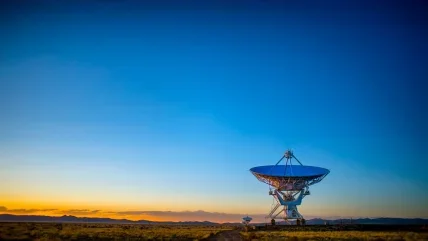
The traditional 20th century way of managing water resources has been mostly interventionist – where we have built physical structures to store, divert and treat water. Most often, the common solution has been to build storage structures, such as dams that create artificial reservoirs to store freshwater during times of excess and release the water during times of shortage.
While such interventionist approaches have provided a more reliable supply of water for satisfying various human needs such as food, energy, flood protection and drought management, they have also come with various downsides. A less documented and understood side-effect of such interventionist approach to water resources development is that of the impact on local and indigenous populations of the developing world. One of the key reasons for this limited understanding is the lack of in-situ water measurement network or a limited network for tracking water resources. This challenge is exacerbated by the remoteness of the region and the complex hydro-politics of data sharing where most entities prefer not to share water data.
Fortunately, if we think of the entire cycle of water, we have actually been tracking most of the components using the vantage of space since the late 20th century. Given that today we are able to track the water cycle and have a long historical record from a source other than ground networks, we can use such satellite remote sensing data to understand the impact of a water resources project on local indigenous populations. Such satellite data are mostly public and global with large spatial coverage, immune to data-sharing hurdles on the ground, and not as affected by on-ground network maintenance and cost challenges.
In this article we summarise what satellite remote sensing data can tell us about the impact on water availability and quality for the indigenous population of the Xingu region in the Amazon due to the Belo Monte Dam project in Brazil. We hope our exploration can motivate other communities to take inspiration from satellite remote sensing that can be the eyes in the sky to decipher the impact of water resources projects on local and vulnerable populations, that is otherwise almost impossible using traditional ground-based approaches.
Indigenous population
The Amazon River basin has a drainage area of more than 60 million km2 spanning nine countries and represents the world’s largest network of rivers (Richey et al, 1989). In addition to exceptional bio-diversity, dense vegetation and abundance of surface water, Amazon is also home to indigenous populations (Blackman and Veit, 2018). Current estimates indicate that there are 2.7 million indigenous people, representing 350 ethnic groups who are living in more than 3000 indigenous territories (Thiede and Gray, 2020).
The local population of the Xingu river of the Amazon basin are one such indigenous group of Brazil living near a part of the Xingu River (Figure 2) called the Big Bend. With the construction of the Belo Monte Dam in 2010, the Xingu River – specifically, the Big Bend portion of the river, which is revered as the ‘House of God’ by the Xingu population, has now dried up by as much as 80%. The reservoir covered about 320km2 of lowlands and forested areas, and estimates about uprooted populations are from 20,000-25,000 (Fearnside, 2020). After construction of the dam and embankments, the filling was complete around 2016. Unfortunately, for a project of this scale, where the end-product was going to be among the largest hydropower structures ever built by humans, the indigenous people whose land and water sources were affected the most were never consulted (Langlois, 2022).
Hereafter, using satellite remote sensing, we show how the Belo Monte Project has altered the water availability and quality of the Xingu River for the local Xingu population. We will also use the terms dam and reservoir interchangeably to refer to the same dam-reservoir system.
Vegetation and water cover
Using the visible and near-Infrared (NIR) bands of the Landsat satellite (missions 7 and 8), we tracked the before and after vegetation cover of the Xingu region by computing the Normalised Difference Vegetation Index (NDVI). Figure 3 shows the gradual decline of vegetation cover by about 7% from the time the Belo Monte Dam project began to the start of filling of the reservoirs. Conversely, there has been a near 2% increase in surface water cover due to the reservoir filling as detected by Landsat using the Normalized Difference Water Index (NDWI) (Figure 4). An interesting observation in using the visible and NIR band is that of the seasonal variations where we found the surface water cover to fluctuate from a high 8% during the wet season to a low 1.5%. However, we also observed the issue of local smoke and fog that often rendered Landsat data unusable by producing suspect values. This is an important issue that any water manager or end-user should be mindful of before applying satellite data at the very granular level in space and time to understand impact.
There are two reservoirs comprising the Belo Monte Project. One is essentially a run of the river reservoir created by the Pimental Dam on the Xingu River right around the Big Bend (Figure 2). The second one is the Belo Monte reservoir artificially created with a canal diversion (Figure 2). Hereafter, we will call them reservoir 1 (Pimental) and reservoir 2 (Belo Monte) in our figures and analysis where appropriate.
The global-scale satellite based digital elevation model (DEM) dataset that is available from the Shuttle Radar Topography Mission (SRTM) was created before the Belo Monte Dam project began. Thus, we can use the SRTM DEM data to capture the complete bathymetry of these two reservoirs and apply them in our understanding of river regulation and storage. Often times, such DEM-based reservoir bathymetry can be quantified in terms of Area-Elevation Curve (AEC) relationship which can then be used to calculate the total amount of storage (storage change) that occurs due to a given elevation (elevation change) in the water surface (Biswas et al., 2021). Figure 5 shows the AEC of the Belo Monte reservoir (reservoir 2) created via the artificial canal to divert the Xingu River flow from the bend (Figure 2). What is evident from this AEC relationship is that the natural topography of the region can accommodate a maximum inundation extent or surface area of about 300 sq. kilometers (Figure 5).
Changes in reservoir storage
Using such an AEC relationship and satellite data on surface water extent (from Landsat) we can compute the storage change patterns of the Belo Monte reservoirs. Figure 6 shows one such variation in storage change for the Pimental Dam (reservoir 1) and Belo Monte dam (reservoir 2). There is however one cautionary tale to be shared here. On close observation, one can notice the sudden drops in surface area for no apparent reason even during times when cloud cover is minimal (Figure 7). As already mentioned, local smoke pockets and fog could be the reason for such artefacts caused by the use of satellite data in the visible and NIR bands that the end-user must be aware of. Fortunately, such issues are being tackled by the community by employing multiple sensors and more robust methods of surface area estimation (Das et al. 2022).
How much water is being diverted?
A common method for tracking how much water a reservoir is diverting or releasing, is to apply storage changes and the inflow in a mass balance. If we ignore all other flux components of a dam such as evaporation (which can be negligible for the Amazonian region) and seepage loss, then inflow is all that we need to know to understand what the reservoirs of Belo Monte dam project are doing to the Xingu River (Figure 8).
Getting the inflow is however a challenge as the Xingu region, to the best of our knowledge, does not have an operational streamflow measuring network at the reservoir inlets that is publicly accessible. There are two ways to mitigate this issue. The first is to use any nearby gauge information with historical flow data and ‘transfer’ that information to the inlet location of interest. The other approach is to apply a hydrologic model forced with satellite data to estimate inflow.
For the sake of simplicity, we show the example of the former method that is widely used in water practice. Here, we have assumed that 70% diversion of Pimental Dam outflow to the Belo Monte reservoir according to de Oliveira et al. (2004) (Figure 8). Figure 9 shows possible outflow scenarios for the two reservoirs. The inflow was derived from gauge data available from the Global Data Runoff Center (GRDC) at Altamira. However, this location had incomplete data for the period of interest and was therefore ‘recreated’ using a more complete record from another location at Boa Sirte and by applying regression analyses. The approach has limitations and will produce significant uncertainty. Nevertheless, it is one of the more feasible ways to derive a first peek into how the reservoirs may be diverting water.
Cooling or warming?
One of the water quality parameters that satellite remote sensing can derive using the thermal IR band is the surface water temperature if the water surface body is wide enough and conditions are clear. Such data from the Landsat mission has already been used extensively in the study of the impact of various dam projects on water temperature in the developing region (Bonnema et al., 2021; Ahmad et al. 2021). One caveat of using such data is that the temperature is not representative of the depth-averaged temperature and may also be influenced by overlying and diurnal variations of the air temperature.
Here, we quantified the time series of temperature of the water surface from Landsat at locations upstream of the Belo Monte Project, on the reservoirs and downstream of the Belo Monte Project. Figure 10 reveals that surface water temperature has not been impacted much after the filling and operation of the Belo Monte Dams. However, one interesting pattern observed is that after 2016 (since the start of dam operations), the spatial variability of temperature along the Xingu River system appears to have lessened. There is a noticeable shift in temperature variations from the natural (pre-dam) fluctuations. Such a finding could have important implications for conservation biologists, freshwater ecologists and communities that depend on the biotic environment for their livelihood needs.
Unprecedented insight
Satellite monitoring can provide unprecedented insight into the operations of dams like the Belo Monte Dam Project and their impact on downstream populations, particularly the vulnerable and local indigenous populations of the Xingu River. In this study, we have shown an example of the insights we can gain from the vantage of space that are otherwise impossible to gain using traditional ground-based approaches. Existing satellite data can be used to monitor recent historical behaviour of a dam’s operations, track the state of the river and pat. vbterns of inflow and outflow at the dam, and even forecast the likely state of the reservoir. Much of that data is easily accessible and free. For example, a tool created for the regional governing body of the Mekong River Commission is now empowering communities along the river in Southeast Asia by giving them access to satellite data about water flow at each dam – data that cannot be hidden or modified by those in power (Das et al., 2022; see http://depts.washington.edu/saswe/mekong).
While estimates based on remote sensing can have higher uncertainty than on-site measurements, unfettered access to such information can provide local populations with evidence to argue for more water releases or make suggestions on how dam projects could be revised to have less negative impact on indigenous livelihood. We hope the approach we have shown here will inspire others to explore the use of satellite remote sensing to decipher the less documented impact of water resources projects on local populations in developing and remote regions.
The authors are Faisal Hossain, Pritam Das, George Brencher, Hannah Conroy, George Darkwah, Andrew McCall, Sanchit Minocha, Geneva Schlepp, Shunyu Yao and Shahzaib Khan. Department of Civil and Environmental Engineering, University of Washington, US.
REFERENCES
Ahmad, S., F. Hossain, G. Holt, S. Galelli, and T. Pavelsky (2020) Predicting the likely thermal impact of current and future dams around the world, Earth’s Future, https://doi.org/10.1029/2020EF001916,
Biswas, N., F. Hossain, M. Bonnema, H. Lee, F. Chishtie (2021). Towards a Global Reservoir Assessment Tool for Predicting Hydrologic Impacts and Operating Patterns of Existing and Planned Reservoirs, Environmental Modeling and Software, vol. 140, https://doi.org/10.1016/j.envsoft.2021.105043
Biswas, N. and F. Hossain (2022) A Multi-decadal Analysis of Reservoir Storage Change in Developing Regions, Journal of Hydrometeorology, vol. 21(1), pp 71-85.
Blackman, A. and P. Veit (2018) Titled Amazon Indigenous Communities Cut Forest Carbon Emissions, Ecological Economics, vol. 153 pp. 56-67, ISSN 0921-8009
Bonnema, M., F. Hossain, B. Nijssen and G. Holt (2020) Hydropower’s Hidden Transformation of Rivers in the Mekong, Environmental Research Letters, https://doi.org/10.1088/1748-9326/ab763d
Das, P., F. Hossain, S. Khan, N. K. Biswas, H. Lee, T. Piman, C. Meechaiya, U. Ghimire, K. Hosen (2022) Reservoir Assessment Tool 2.0: Stakeholder driven Improvements to Satellite Remote Sensing based Reservoir Monitoring, Environmental Modeling and Software, vol. 157. https://doi.org/10.1016/j.envsoft.2022.105533,
de Oliveira CARVALHO, N., Arruda CAFÉ, F., de Oliveira MOTA, G., Costa de Barros FRANCO, H., Eng, C., & -Centrais Elétricas Brasileiras, E. S. (2004). Assessment of the Sedimentation in the Reservoirs of the Belo Monte Hydroelectric Complex, Xingu River, Brazil. https://www.iahr.org/library/infor?pid=17352
Fearnside, P. M. (2017). Brazil’s Belo Monte Dam: Lessons of an Amazonian resource struggle. DIE ERDE – Journal of the Geographical Society of Berlin, vol. 148(2-3), pp. 167-184. https://doi.org/10.12854/erde-148-46
Langlois, J. (2022, January 13). An Amazon Defender Stands Up for Her Land and Her People. Yale E360. https://e360.yale.edu/features/an-amazon-defender-stands-up-for-her-land-and-her-people
Thiede, B. C and C. Gray (2020). Characterizing the indigenous forest peoples of Latin America: Results from census data, World Development, vol. 125, ISSN 0305-750X, https://doi.org/10.1016/j.worlddev.2019.104685.
Richey, J.E., C. Nobre and C. Deser. (1989) "Amazon River discharge and climate variability: 1903 to 1985." Science, vol. 246, no. 4926, 6 Oct. 1989, pp. 101